By its very nature, biomimicry is a transdisciplinary practice, and the Biomimicry Center’s research goals align with the New American University’s aspirations to conduct “use-inspired research” for positive societal impact.
At the Biomimicry Center, we build collaborations and promote biomimicry research across campus. We work with researchers from different departments to develop project ideas and collaborate on Broader Impacts components of grant proposals. We build the skills of our students to be engaged in real-world projects on biomimicry. Through our partnership with Biomimicry 3.8, we also help engineers and design teams connect with relevant biology labs on campus and external resources and contacts.
If you’re interested in discussing how biomimicry and The Biomimicry Center might play a role in your research efforts and/or a grant opportunity you’ve identified, please contact us.
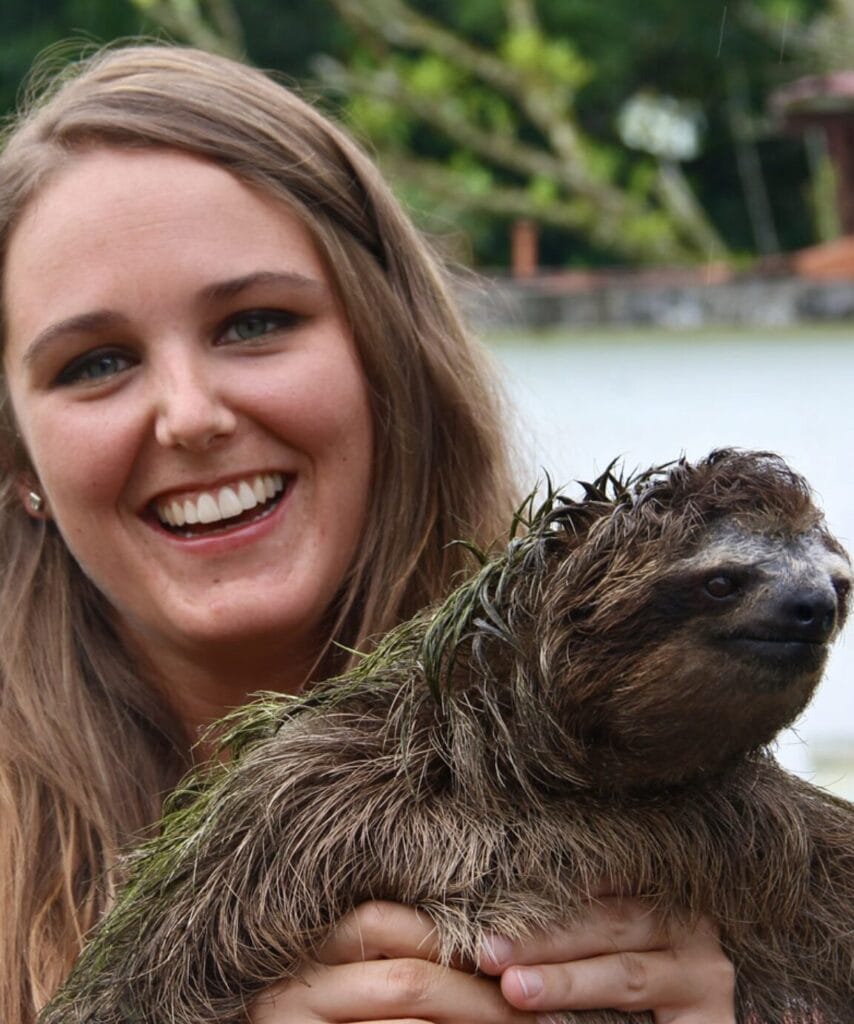
Lab to market
Translation of biomimicry and nature-inspired knowledge from the lab that is brought to the market.
Biomimicry in Action (BiA
A laboratory that brings together students and interested organizations to imagine innovative and regenerative solutions to real-world problems.
Biomimicry in Action (BiA)
Through this program, organizational partners, in collaboration with The Biomimicry Center at (ASU), engage with students in a mutually beneficial technical relationship. Challenges framed by the organization are addressed by teams of specially selected undergraduate and graduate students working with guidance from faculty mentors and external advisors. For students, the program provides an opportunity to apply their skills and knowledge of biomimicry, sustainability, and other fields of expertise they bring to the table to explore a complex, team-oriented project, typically completed over one semester. For the external partners, the project provides multiple opportunities: a cost-effective approach to address technical problems through an interdisciplinary team selected across ASU and the ability to interact with students to gain access to fresh ideas and thinking and to mentor potential future talent. Below are some examples of previous projects.
Biomimicry in Construction Practice
Working in consultation with Biomimicry 3.8, the world’s leading bio-inspired consultancy, the BiA team developed new standards and best management practices (BMPs) in construction & land management by using biomimicry Life’s Principles. The team was composed of two undergraduate students in biomedical and mechanical engineering and two graduate masters of biomimicry students with a background in construction management and aerospace engineering.
Circular Economy and Diapers
Working with a global consumer products company interested in developing nature-inspired solutions to diaper waste and mimicking nature’s cyclic processes, a team of five undergraduate and graduate students with specialties in biomimicry, business, materials, supply chain, and sustainability ideated around a dozen bio-inspired models for circularity of diapers and produced the framework for a pilot currently underway in South Africa.
For more information, click below.
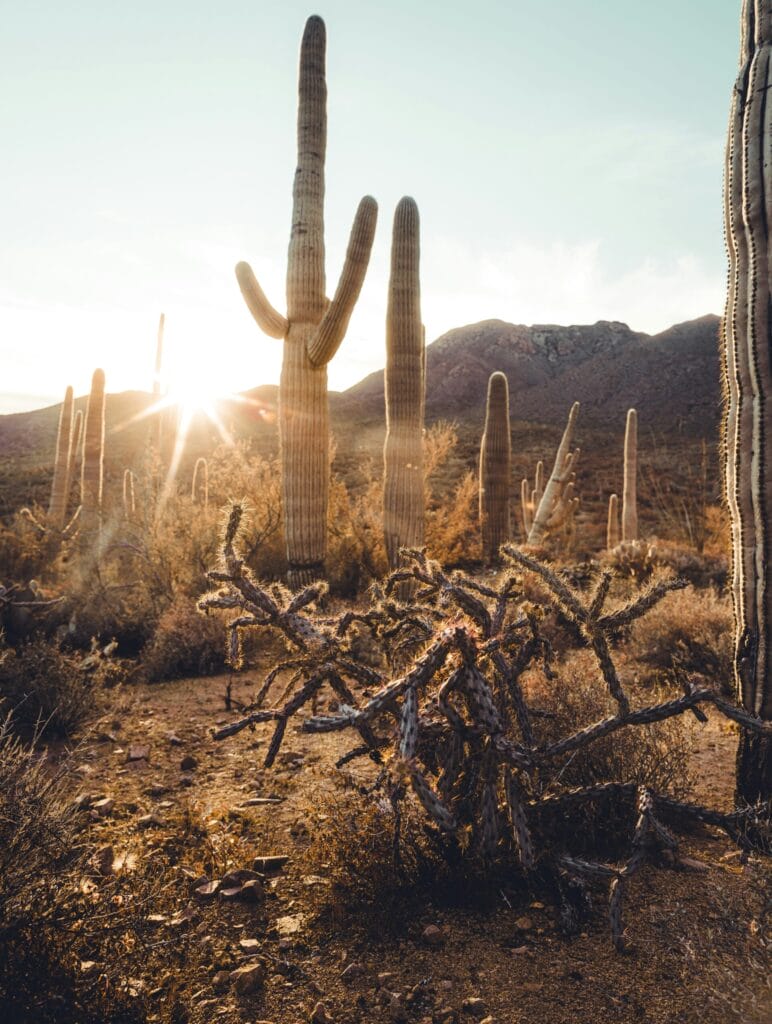
Previous Seed Grants
For five years, the Biomimicry Center supported innovative biomimicry projects for interdisciplinary teams of ASU faculty. Projects ranged from creating bio-inspired innovation in the design of products, buildings and urban systems to exploring biological models that could inspire new technologies. Researchers from any school or department at ASU were encouraged to apply. Below is a sample of our projects.
2018
Investigators:
Dhruv Bhate1, Christine Lee2, and Lara Ferry3
1The Polytechnic School, Ira A. Fulton Schools of Engineering, Arizona State University, Mesa, AZ
2School of Art, Herberger Institute for Design and the Arts, Arizona State University, Tempe, AZ
3School of Mathematical & Natural Sciences, Arizona State University, Glendale, AZ
Additive Manufacturing is increasingly enabling the incorporation of cellular structures, such as honeycombs, foams and lattices, in the manufacturing of functional parts with reduced material utilization and improved performance-to-weight ratios. A key challenge, however, is the high uncertainty of the integrity of these materials due to their heterogeneous structure. Proliferation of cellular materials in engineering structures is likely to require a step-change in design thinking. One such paradigm shift that we seek to explore is the use of asymmetry in constructing cellular materials, and how this may benefit overall structural integrity. The inspiration for this work comes from the venation of insect wings, which survive a wide range of loading conditions and demonstrate large diversity in patterns across species with varying local asymmetries. Within species, however, these patterns are so consistent that they are reliable taxonomic and phylogenetic characters, suggesting convergence on a functionally favorable form. The investigators will combine their expertise in functional morphology, additive manufacturing, and artistic and engineering design to abstract design principles from insect wing venation patterns, and use Additive Manufacturing to identify those that lead to improvements in resilience of engineering structures.
Nicholas Stephanopoulos1, Chris Diehnelt2, and Sarah Stabenfeldt3
1School of Molecular Sciences & the Biodesign Center for Molecular Design and Biomimetics, Arizona State University, Tempe, AZ
2Biodesign Center for Innovations in Medicine, Arizona State University, Tempe, AZ
3School for Biological and Health Systems Engineering, Arizona State University, Tempe, AZ
We propose to create “nano-synbodies”: synthetic nanostructures that mimic antibodies in their ability to bind a target with high affinity and specificity. Antibodies achieve their exquisite targeting abilities by using a protein scaffold to position three variable peptide loops in a confined nanoscale volume. Here, we will develop antibody mimics from both top-down and bottom-up approaches. For the bottom-up approach, we will use a peptide array to discover 20 low-affinity peptides for a protein target. These peptides will be synthesized with single-stranded DNA handles, allowing them to be positioned on a DNA nanoscaffold bearing complementary handles. We hypothesize that some combination of peptides will yield structures that bind to different faces of the protein, so systematically testing all 1,140 possible trivalent combinations will allow us to find nano-synbodies that bind with high affinity and specificity. The exact nanoscale geometry of the DNA scaffold can be varied to tune the presentation of the peptides, and we will investigate structures, such as a six-helix bundle, tetrahedral cage, 3-way junction, or a DNA origami with a triangular cavity. The second top-down approach will use known antibody CDRs identified through phage display to recapitulate the specificity and affinity of recombinantly produced proteins.
Konrad Rykaczewski1 and Gordon W. Schuett2
1School for Engineering of Matter, Transport and Energy, Arizona State University, Tempe, AZ
2Department of Biology, Georgia State University & the Chiricahua Desert Museum, Rodeo, NM
Finding better routes of harvesting water vapor and droplets is critical to improving efficiency of clean water production (e.g., fog and dew collection, thermal desalination) and power generation methods. While many bioinspired concepts that rely on altered wettability patterns and static morphological features have been described (e.g., based on horned lizards, beetles, pitcher plants, and cacti), the PIs want to explore potential benefits of coupling wettability patterns with adaptive morphological changes. Specifically, the PIs propose to study the rain harvesting behavior of western diamondback rattlesnakes (Crotalus atrox). Through the unique synergy of biological and engineering expertise and resources, the PIs will study both the macroscopic and the microscopic aspects of this process. The research will be performed under the PIs supervision by interdisciplinary undergraduate and graduate students at ASU. The results of the study, which will be disseminated through conference presentations and journal publications, will provide preliminary data for seeking external
See publication: Role of Scale Wettability on Rain-Harvesting Behavior in a Desert-Dwelling Rattlesnake
2016
Konrad Rykaczewski1 and Lucas C. Majure2
1School for Engineering of Matter, Transport and Energy, Arizona State University, Tempe, AZ
2Desert Botanical Garden, Phoenix, AZ
The Sonoran Desert has an incredible diversity of cacti, which possess equally diverse numbers of special properties. Strong water repellency is one of these special characteristics. The stems of some of these cacti, such as Opuntia, also known as prickly pear, possess superhydrophobic characteristics due to a waxy surface with hierarchical nanoscale and microscale texture. While watering a growing collection of Opuntia microdasys in his yard, however, the PI observed a striking difference in water repellency and wetting in individual prickly pear plants. Recently formed and grown parts were indeed superhydrophobic, but the older stems located near ground were superhydrophilic. To the best of our knowledge, this age- and location-dependent variation of wetting properties and its function have not been described. The PIs want to combine their expertise in surface science and the evolutionary biology of cacti to systematically explore how surface texture and composition, as well as wetting properties of Opuntia microdasys, change with age and how these changes might offer advantages for the plants’ survival. In parallel, the PIs will explore how this idea could be mimicked to achieve smarter water-manipulation techniques for applications ranging from more efficient water capture to decontamination.
See publication: Microscale mechanism of age dependent wetting properties of prickly pear cacti (Opuntia)
Diego García Setién Terol1
1The Design School, Herberger Institute for Design and the Arts, Arizona State University, Tempe, AZ
The Air Tree was an essential component of a pilot project known as the Ecoboulevard, built in Madrid, Spain, between 2004 and 2008. The purpose of these treelike structures, which were inspired by ideas from plant forms and physiology, was to provide bioclimatic conditioning of open-air public spaces. This project will explore how the urban Air Trees of Madrid could be adapted for use in the desert city of Phoenix, Arizona. The project will update the original technology and components (PV panels, fans and nebulizers, lighting systems, etc.) of the Air Trees as well as look to the native species of the Sonoran Desert for inspiration in creating new place-based forms and functions for these structures. Explorations include examining how the Air Tree could mimic the self-shading shapes of some cactuses, like those from the Carnegiea and Ferocactus genera, or the arrangement of fluid conduits around the cactus stem. The expected outcome is to create a more efficient form factor of the Air Tree for Phoenix’s environmental conditions.
Ximin He1
1School for Engineering of Matter, Transport and Energy, Arizona State University, Tempe, AZ
The efficient extraction of molecules from fluid mixtures is vital for applications ranging from chemical analysis in water treatment to toxic or rare metal removal and recovery. But current methods are less energy efficient, relying on high-energy supply and multi-step operation. Inspired by biological processes that seamlessly synchronize the capture, transport and release of biomolecules, we propose to use smart polymer (stimuli-responsive hydrogel) to build adaptively reconfigurable material to achieve concerted “catch and release” of target molecules. We expect to apply the biological system’s strategy to create a new class of bioinspired, autonomous chemical separation material systems. The new separation material system will, for the first time, demonstrate the utilization of cascade, chemo-mechanical energy transduction inherent in smart materials to control and automatize sequential adsorption-desorption, leading to one-step metal recovery without significantly enhanced separation rate and energy efficiency. The systems will be low-cost, environment-friendly, and encompass significant modularity and design flexibility to permit integration and upscaling for broad applications. The proposed technology will suggest great promise for chemical engineering (sugar, food processing), environmental engineering (water treatment, rare metal recovery, and spent nuclear waste extraction), biomedical engineering (protein, cell, bacteria, and drug separation), preconcentration for downstream analysis with low turnaround times.